Geology of the Laurels
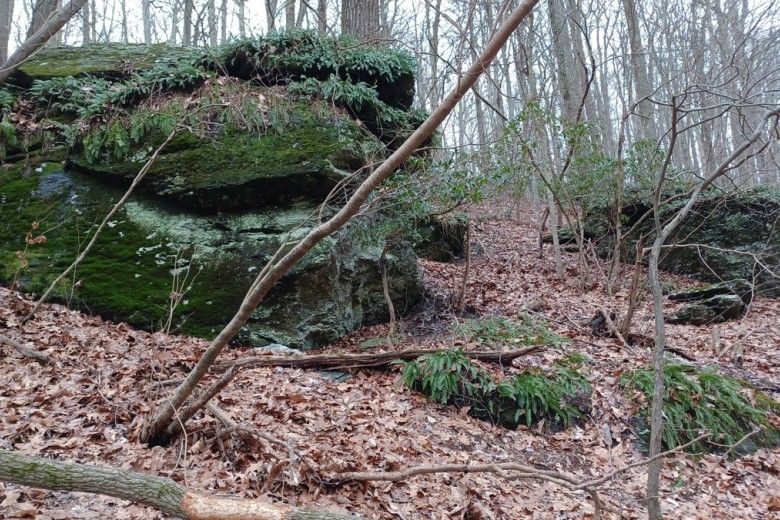
Rocks are literally the foundation of the Laurels. They provide a firm footing to trails, jut in dramatic outcrops along hillsides, and furnish steps to cross streams. Unseen, rocks underlie the Preserve’s hills and valleys. In addition to adding beauty, rocks tell stories: the history of continents and ancient seas, and how landforms continue to change today. But you have to know how to read and understand the language of rocks.
One could not ask for better translators than LeeAnn Srogi and Tim Lutz, both emeritus Professors in the Earth and Space Sciences Department of West Chester University. They shared their expertise with me as the basis for this brief guide.
Setting the scene
Walking the main trail (McCorkles Road) through the Laurels from the parking area, along Doe Run and Buck Run to its end, you will notice ledges of dark, layered rock—some large enough to extend like sheltering roofs. You’ll also see smaller flattish rocks scattered on the slopes, and platelike rocks on stream bottoms. Several trails branch off the main trail to the east, climbing stream valleys with similar rock features.
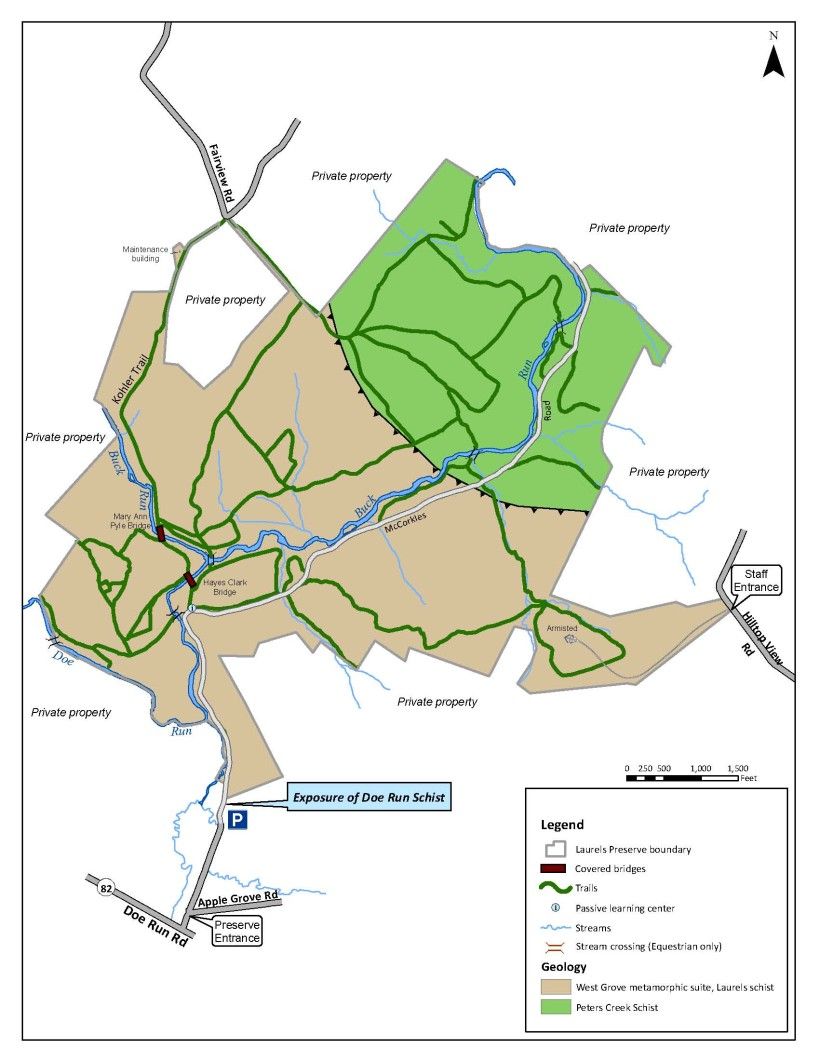
This map shows the bedrock underlying the Laurels. There are two kinds of rock within the boundaries of the Laurels, both a type of schist: Laurels Schist and Peters Creek Schist. Geologists refer to each rock type as a “unit,” named after the place where it was first identified and described in detail.
Schist is a metamorphic rock. Metamorphic rocks were the last of the three rock types to be understood by geologists. Igneous rock is formed when molten rock (magma) or volcanic lava cools. Sedimentary rock is formed when particulate material such as mud, sand, gravel, or clay, or organic material such as shells or plants, are compacted and cemented together (“lithified,” to use the technical term). Metamorphic rocks are created when igneous or sedimentary rocks are transformed under immense heat and pressure; in the case of schists, the original rocks were mudstone (shale) and sandstone. These sedimentary rocks were squeezed and heated to the point where the original minerals that made up the sediment were replaced. But what was the source of all that heat and pressure? And where did those sedimentary rocks come from in the first place?
To answer those questions we need to go back in time.
Return with me to those thrilling days of yesteryear
The geologic time segment is the early to middle Cambrian period, 570-475 million years ago (“Ma” in geo-shorthand), the earliest part of the Paleozoic era. During that time, the area that is now the Laurels was at the bottom of the Iapetus Ocean. This warm, shallow sea started to form about 600 Ma when an ancient supercontinent, Rodinia, broke into continent-sized pieces. As two of these, Gondwana and Laurentia, drifted apart, the ocean filled in the gap (which geologists term a “rift”). Laurentia is the ancestor of North America. Confusingly enough, Laurentia and Iapetus were located around the Equator and Laurentia was oriented east-west rather than its current north-south. But we will use the current direction for clarity’s sake.
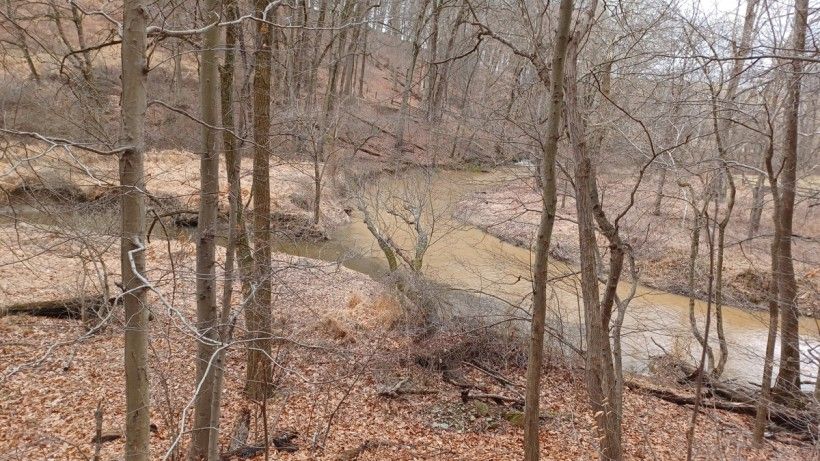
The Laurentian highlands to the west of the ocean were eroded by running water, and in the same way that Buck Run and Doe Run transport sediments eroded from the Laurels hills, these sediments were carried into the ocean. Depending on the origin of the sediments, they were either mud or sand. Geologists refer to such sediments as “clastic.” These may form layers on the seafloor as smaller particles settle above larger ones after storms or where rivers mingle.
Meanwhile the ocean itself produced a build-up of non-clastic sediments on the reef-like bottom. These were “carbonates,” the calcium carbonate remains of marine invertebrates. The uneven ocean floor contained local high and low points. Carbonate sediments precipitated from seawater in sun-warmed, shallow areas.
These clastic and non-clastic sediment deposits occurred over a long period of time, during which the continental plates holding Laurentia and Gondwana (which corresponds to western Africa) were moving further apart.
Then, the plates changed direction. Starting about 500 Ma Laurentia and Gondwana began spiraling toward each other, like two ballet dancers; Laurentia also began changing its orientation to north-south. Between 450 and 410 Ma a portion (terrane) of Gondwana called “Ganderia” broke off in a northwest spiraling movement and collided with Laurentia. As Ganderia neared Laurentia, forces from the plate motions caused smaller terranes—fragmentary micro-continents caught up in the collision—to pile up on top of each other “like a stack of overlapping pancakes or playing cards,” in Dr Srogi’s analogy, along the edge of Laurentia. The collision also resulted in the uplifting of mountains to the west, in the Salinic and Acadian orogenies.
At a “subduction zone” where the two continent-size rock masses collided, they slid along, over and under each other in complex ways. Rocks along the Laurentian edge were subject to intense heat, compression and stretching. The result, in a word, was metamorphism.
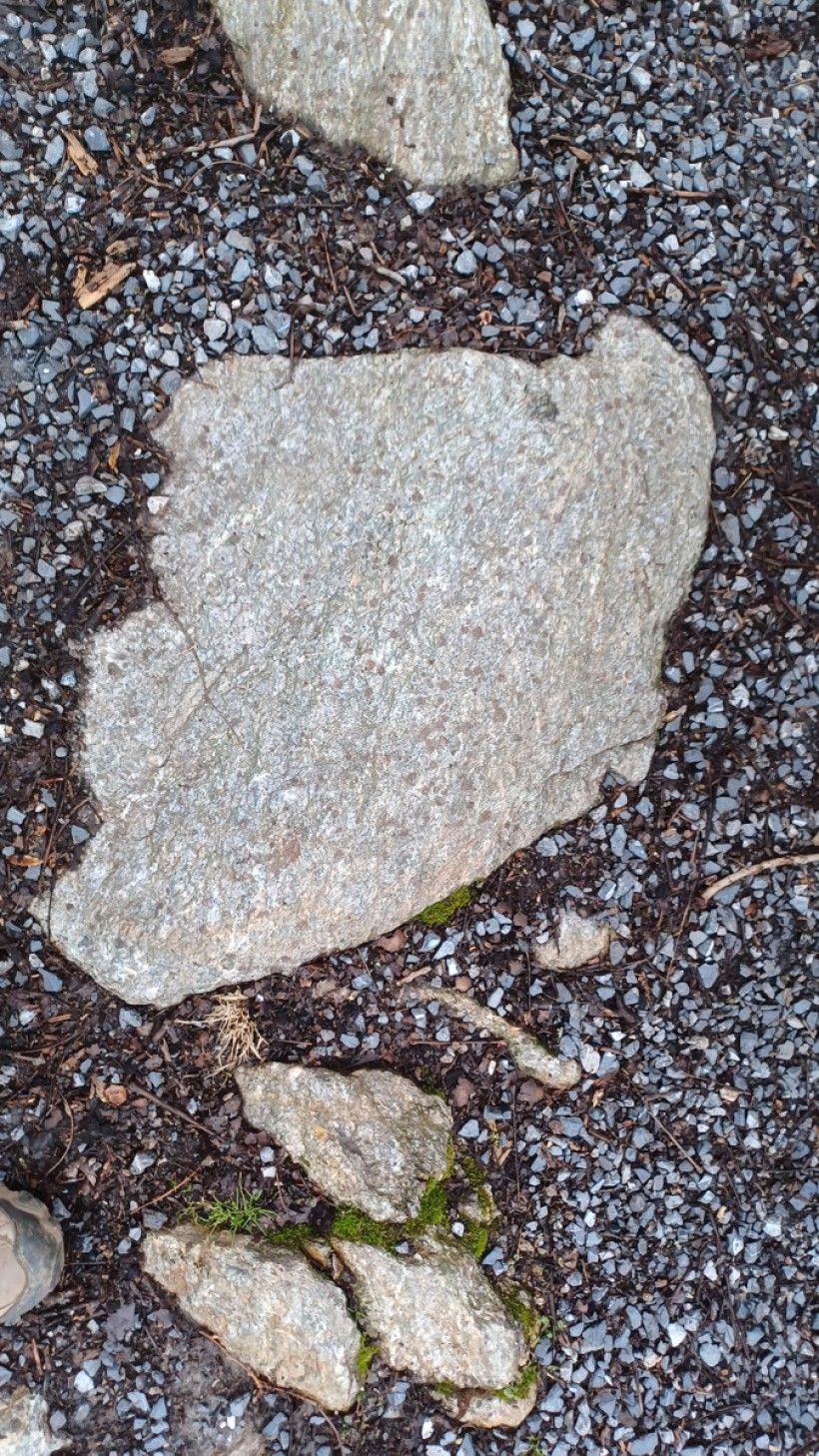
Schist, schist and more schist
The metamorphism of the sedimentary rocks along the collision zone caused the original minerals to be altered to different minerals. For example, clay (argillaceous) minerals in rocks such as shale are transformed into muscovite, chlorite and biotite: all lustrous, flaky mica minerals whose color ranges from black to clear, depending on the chemical composition. (Chlorite is not chemically a mica, but looks enough like one that we’ll include it in the extended family for this guide.)
The platy physical character of these minerals results in the formation of foliated rock, that is, with many thin layers like pages in a book, as crystals line up and rotate perpendicular to compressive forces.
This foliated rock is referred to as slate. If it continues to be subject to metamorphism it is further transformed into phyllite, then schist and finally gneiss. By the time the rock has become gneiss it has lost its platy character; gneisses are granular. The name “schist” is derived from the Greek word for “split,” since the rock splits easily along the layers.
In addition to the various micas, two other typical components of schist are quartz and feldspar, which are silicate and aluminum silicate minerals, respectively. Feldspar in schist occurs in a striated form called plagioclase. Other common schist components are staurolite (an iron-aluminum silicate with star-shaped crystals), kyanite (an aluminum silicate), and epidote (a hydrous silicate).
Another common component of schist is garnets, which are dark-colored silicate crystals that form under certain metamorphic conditions. They may be quite large and conspicuous. Garnets in the schists found at the Laurels are deep-red to red-brown.
The chemical components of the original rocks (protoliths), and whether temperature or pressure was increasing or decreasing at the time of metamorphism, are key factors determining the mineral composition of the metamorphic rock. At the same time, though, the heated compressed rocks are being squeezed, twisted, rolled and stretched. As Dr. Srogi explains, they are “like Silly Putty” during metamorphism. The directions of kinetic forces (the sliding, twisting, and so on) affects the morphology or shape of the crystals and determine whether minerals are aligned to form foliation.
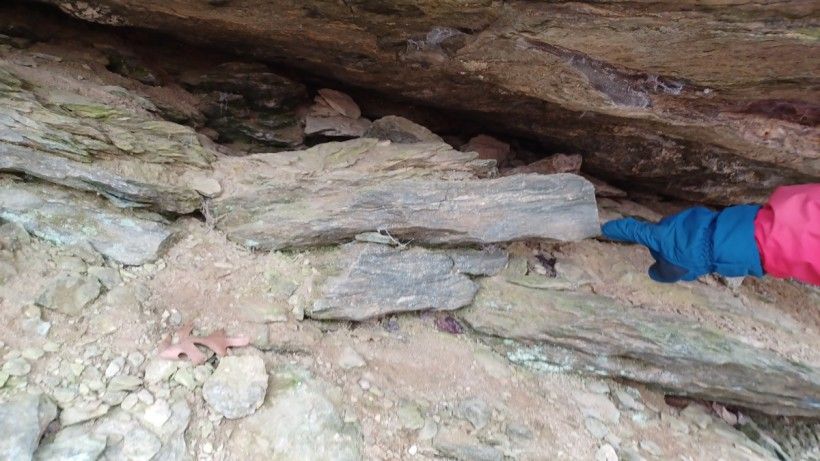
Since schists can be composed of many different minerals, they are differentiated by using the predominant minerals. These in turn enable differentiation by typical coloration. So Laurels schist is silvery-gray-green because it has lots of chlorite and muscovite, as well as some plagioclase, and may have quartz veins. Peters Creek schist is very similar in appearance but has more quartz. It may have pinstriping due to thin layers of mica and “metasandstone” (metamorphosed sandstone). Neither of these schists typically has garnets. Laurels Schist was formed from sediments with much more mud than sand; Peters Creek Schist sediments had more sand than mud and were probably deposited earlier and in a different location.
The Embreeville Fault
So, now we know how the schists were formed and what distinguishes them mineralogically. What else can we tell from the way these two schists occur in the Laurels?
Referring again to the map, the schists occur in two curving bands. Between the Peters Creek Schist on the northern end of the Laurels, and the Laurels schist, the map has a dark black curvy line with sawteeth triangles pointing away from the Peters Creek schist. The dark band is the inferred location of the Embreeville Fault.
A fault line is a line of displacement where two rock blocks have moved relative to each other because of the tectonic movements and collisions of plates, which cause fracturing and movement at very large scales. This movement probably occurred as a result of the collision of Ganderia and Laurentia described earlier.
Fault movement can be lateral (one plate moves alongside the other) or vertical (one moves up or down relative to the other). The Embreeville Fault is the latter type, and is a “thrust fault”, where one rock block moved at an angle, up and over the other.
The Embreeville fault occurs where the deeper, hotter rock unit containing Laurels Schist moved up and over the cooler Peters Creek schist. (The sawteeth triangles on the map show the direction of movement and overlap of strata.) This is the result of the collision of the Ganderian terrane with the Laurentian plate.
Geologists are able to deduce this history from laboratory work on the rocks, especially through electron microscopy and radioactive isotope analysis.
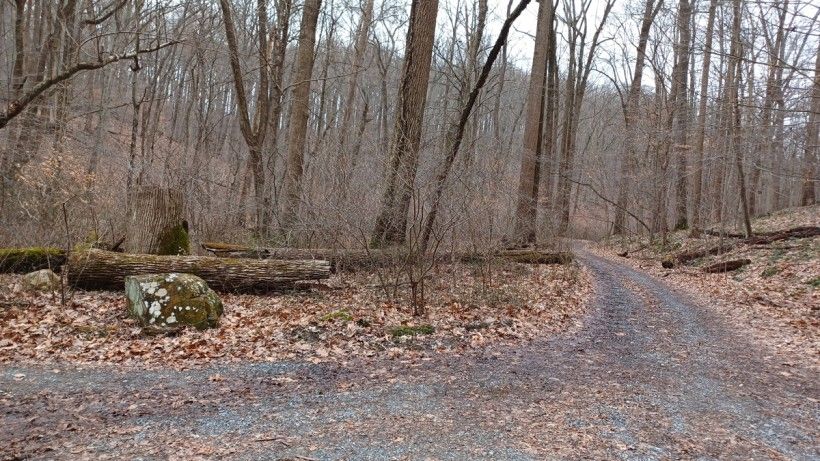
However, note that I referred above to the “inferred” fault line. Walking in the Laurels, you will not see any evidence of the fault itself (other than what can be inferred from the inverted schist layers). As indicated on the map, the fault crosses McCorkles Road approximately 1.25 miles north of the parking lot, in a wide stream valley (where there is the turnoff to the trail to Armisted). If you didn’t know that there was a fault line here you would not think anything of it. Indeed, it is hard to imagine how deep underground these schist layers were when they were squeezed and displaced: 10 to 20 miles below the surface of the earth. Of course, this incredible depth is why the rocks were subject to such heat and pressure. The rocks that were on top of the schist just below our feet have eroded away.
Now where were we…
Now that we know the backstory, let’s return to our walk.
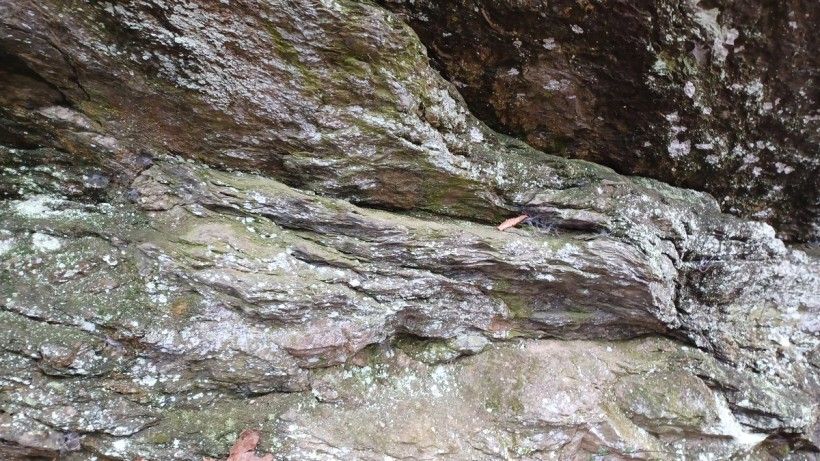
Entering the Laurels on McCorkles Road, we begin in the zone of Laurels Schist. There are many exposures of Laurels Schist along the trails. It has smooth surfaces, with distinct layers of quartz-feldspar and mica layers. Viewed on edge, as in the large outcrops along the trail, Laurels Schist looks wavy. This twisted appearance is evidence that the rocks were moving while also being squeezed during metamorphism and that the tectonic forces changed direction over time.
Large rock outcrops pepper the hillside. Some are large enough that they are physically connected to the underlying bedrock, but most of the boulders have moved downhill over time.
Continuing on the trail, we reach a wide valley where a spur trail goes uphill to the southeast toward Armisted. Here, we pass imperceptibly across the Embreeville Fault, into the Peters Creek Schist zone. Peters Creek Schist is not exposed right at the fault, but there are outcrops along McCorkles Road trail as it continues north. This rock weathers to a greenish cast, and often contains layers of “metasandstone,” which look like the original sedimentary rock although it has been metamorphosed.
Beyond the Laurels
Just outside the Laurels proper, three other kinds of rock deserve note. (For a map that shows the larger geological context, see the Coatesville quadrangle, referenced below.) Immediately south of the parking area, the entrance road traverses a band of Doe Run Schist. There are prominent exposures of this rock along the roadside. Doe Run Schist is gray-black with lots of silvery mica, and “chunky” in texture (geologists would say “coarse-grained”). There are often red garnets embedded in the surface.
Doe Run Schist is thought to be the same rock unit as Laurels Schist; Laurels Schist is a sub-unit of Doe Run, which occurs in a wide band in our region. The two look different and contain different minerals. Doe Run is coarser-grained and has much less chlorite; it is mostly silvery muscovite, and typically has visible crystals of garnet, staurolite, and kyanite. By comparison, the Laurels Schist is finer-grained with much chlorite and muscovite, but no garnets. Laurels Schist only occurs in a narrow band along the Embreeville fault. It was created as a result of localized metamorphism when the hotter Doe Run Schist slid over the cooler Peters Creek Schist, causing hot watery (hydrothermal) acidic fluids to be released into the Doe Run Schist that altered its minerals. (This is why the description of the Embreeville Fault above referred to the rock unit containing Laurels Schist, since it could more accurately be described as Doe Run/Laurels Schist.)
Between Doe Run Schist and Doe Run Road is a band of Cockeysville Marble, which is a metamorphosed carbonate (sedimentary) rock. This rock was originally formed from deposits at the bottom of the Iapetus ocean and metamorphosed in the same tectonic events as the schists. It is a white, sparkly rock that is infrequently observed above the surface because it rapidly weathers from exposure to air and water. There is a large slab of Cockeysville marble next to the Hayes Clark covered bridge, and blocks of marble in the wall, which were quarried and transported here.
North on Buck Run, past the Laurels boundary and on private property, is a very unusual type of rock. It is an ultramafic rock, serpentinite. This metamorphosed igneous rock is high in magnesium and iron (thus ultramafic). Commonly called “serpentine”, the rock is rarely exposed in eastern North America. There is a concentration of exposures in southeast Pennsylvania. This rock was originally bedrock under the seafloor; it was metamorphosed during a tectonic event, but geologists are not sure which one. (“The geologic history of the Pennsylvanian Piedmont has been thoroughly studied, but the origin of the ultramafic bodies remains a source of contention.” (2017 Kerrigan)).
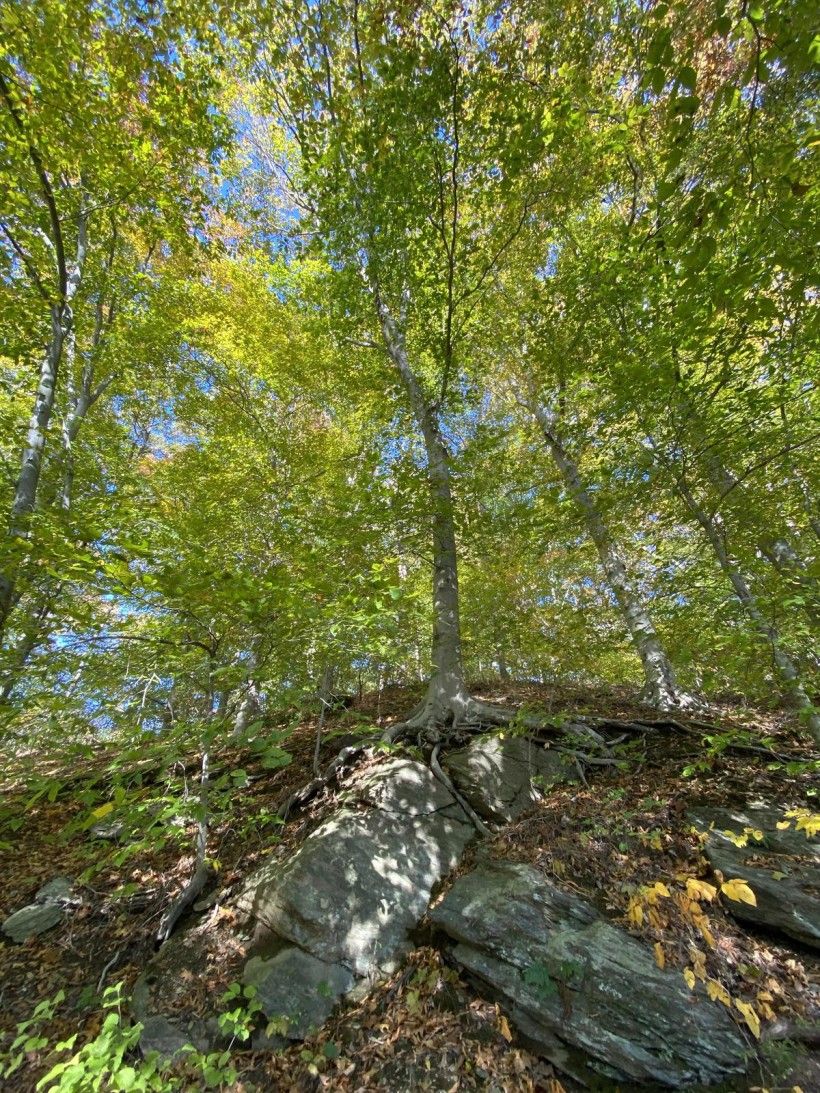
A Rocky History
The history of the understanding of Laurels geology is as fascinating–and complicated–as the geology itself. Let’s start by traveling back in time…not quite as far back as the Cambrian era. In 1902, Florence Bascom published a survey article describing the Wissahickon Formation of mica-schist and mica-gneiss as a rock formation extending throughout the Piedmont of southeastern Pennsylvania, northern Delaware and northern Maryland. She named it for exposures along the Wissahickon Creek.
Bascom (1862–1945) was a groundbreaking figure in the history of US Geology. Only the second American woman to earn a Ph.D. in geology in 1893 (and the first woman to earn a Ph.D. in any field from Johns Hopkins University), Bascom was the first woman hired by the U.S. Geological Survey in 1896. She achieved many honors in her career and made fundamental contributions to the understanding of geological history of the Appalachian Piedmont. The USGS named its Geoscience Research Center after Florence Bascom.
She also founded the geology department at Bryn Mawr College, training many women who became prominent geologists. Among her students were Anna Jonas and Eleanora Bliss. These two women earned a joint Ph.D. in geology from Bryn Mawr; their dissertation was on the relationship of the Wissahickon formation to rocks in the Doe Run-Avondale region. In later work they disputed Bascom’s conclusion that the Wissahickon was of Ordovician age; they identified a “Glenarm formation” of pre-Cambrian age that included the Wissahickon formation.
In the century after Bliss and Jonas challenged their mentor’s conclusions, the structural geology of the Piedmont continued to be controversial, as new research upended prevailing theories in the same way that shifting tectonic plates transformed rock layers. In 1989 two distinct rock accretion zones (terranes) were identified on either side of a fault (later named the Rosemont shear zone). In the mid-1990s the term “Glenarm Wissahickon” was adopted by the Pennsylvania Geologic Survey to distinguish schist and gneiss in formations occurring west of this line. In the last 20 or so years, geologists have developed a new understanding of the Piedmont in southeastern PA and northern Delaware, based on research by Howell Bosbyshell of West Chester University, Gale Blackmer (director of the PA Geological Survey), Sandy Schenck (formerly of Delaware Geological Survey), and Professor Srogi.
The current conception of the Piedmont identifies a “West Grove metamorphic suite” (WGMS) of rocks that includes the Laurels Schist, occurring west of the Rosemont shear zone, formerly named “Glenarm Wissahickon.” (“Glenarm Wissahickon” still appears on standard maps, though, which have not been updated.) The Rosemont shear zone extends in a northeast-southwest direction to the south of the Laurels, intersecting the Delaware state line due south of West Chester, and PA Route 3 at approximately Newtown Square.
The rationale for dropping “Wissahickon” from the name is that recent research shows these two rock formations to be of different ages and origins, and that they were metamorphosed at different times. “[West Grove Metamorphic Suite] is an early Cambrian, Laurentian rift-related sequence and … the Wissahickon Formation was deposited in an arc-related basin during the Ordovician.” [Bosbyshell et al 2014] Further, ”The Rosemont Shear Zone (RSZ) is the boundary between arc rocks and the Laurentia margin.” [Bosbyshell et al 2017] That is, the sediment that became Wissahickon rock, east of the Rosemont shear zone line, was deposited later (Ordovician, as Bliss and Jonas argued) than that of the rock west of the Rosemont line (Cambrian, as Bascom maintained) that became WGMS rock. Moreover, while WGMS sediment was deposited at the edge of the Laurentian proto-continent when the Iapetus sea was rifting apart, Wissahickon sediment was eroded off an arc-shaped chain of volcanic islands that eventually collided with Laurentia. The Wissahickon formation was metamorphosed mainly in connection with a later tectonic event in the Devonian era around 390 Ma, compared with the WGMS which was metamorphosed earlier (~410 Ma). And it appears that the crustal block that includes the Wissahickon formation was moved to its present location from as far away as New England.
Bliss and Jonas raised awareness of the importance of Paleozoic-age thrust faults in the region. It took researchers many decades to recognize the Rosemont shear zone and work out the complex sequence of deformation events.
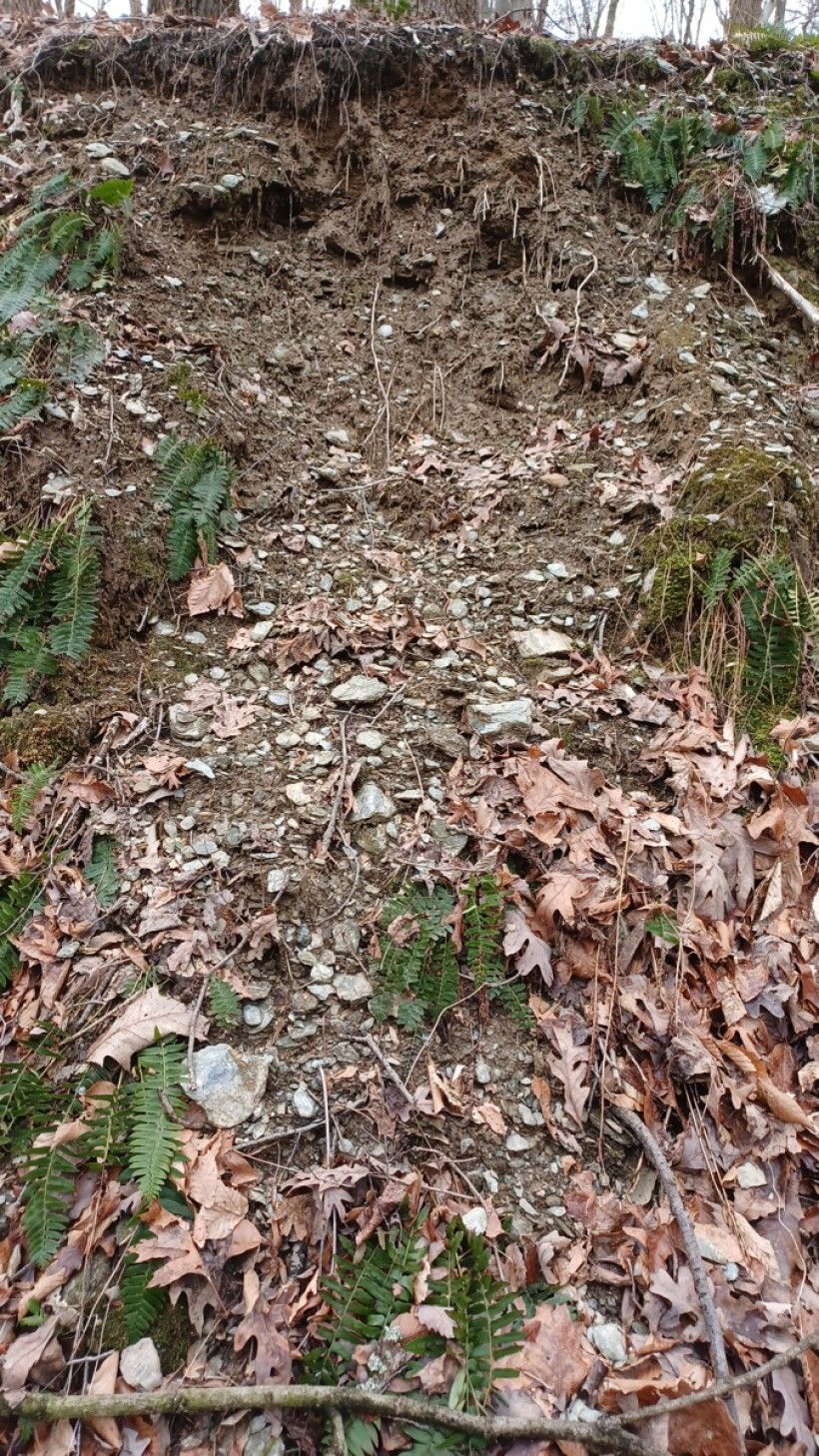
The story continues
After the tectonic events that created and moved Doe Run Schist along the Embreeville fault, Gondwana and Laurentia continued moving together, eventually forming the super-continent Pangea about 300 Ma. Pangea began breaking apart about 250 Ma, eventually resulting in the creation of the Atlantic Ocean and the continents we know today.
Since that time what has happened, geologically, in the Laurels has been the slow and steady conversion of overlying rock to sediment by erosion, and the transport of sediments by water into the ocean, enabling us to walk beside rocks that once were buried as far from the surface as the Delaware Bay is from Doe Run and Buck Run.
Sediment erodes from present-day surface rocks at the Laurels and combines with organic material to become soil, creating landforms: the hills and valleys, bluffs and flatlands of the preserve. This soil, too, is eroding. You can see the process occurring in one of the many “slumps” where pieces of steep hillside break off and slide downward. These processes occur naturally in steep valleys and are exacerbated by human activities such as road construction. You also see the result of erosion after a rain event when Doe Run and Buck Run turn muddy from soil washed into the streams. And you can see soil being deposited alongside the stream in alluvial flats as the stream meanders across the land, first cutting in then leaving sand and gravel as it changes course. These same processes are frozen in time by the rocks in the Laurels. Will our time period someday be studied by future life forms, the geologists of 100 million years from now?
Sources for further study
Bedrock Geology of the Coatesville Quadrangle (Gale Blackmer, 2004)
The Geological Story of Pennsylvania (Barmes & Sevon, 2002)
The Piedmont: Old Rocks, New Understandings (Bosbyshell, et al 2017)
Defining the West Grove Metamorphic Suite (Bosbyshell, Blackmer, Schenk and Srogi, 2014)
Petrology and Geochemistry of the Bells Mill Road Ultramafic Body (Kerrigan et al, 2017)
Geological Origins of the Ridley Creek Valley Landscape and Its Influence on Human Land Use (Cressler, 2020) (while focused on the Wissahickon formation this article includes a very accessible summary of geologic history of southeastern Pennsylvania).
Amazing animations of plate tectonics - and in particular see Precambrian Animation and Plate Tectonics 600Ma to Today